An international team of scientists, including a physicist from the University of Tartu, has made significant progress in the understanding of the dynamics of crystal vibrations of laser-excited tungsten. The results could, among other things, be beneficial in the fields of nuclear energy science and space technology, where computational methods could partially replace the need for complex experiments and fundamental research.
According to Artur Tamm, associate professor of Computational Physics at the Institute of Phymasics, the new modelling methodology can be used to study and develop new materials in several key areas. “Understanding the non-equilibrium effects of laser excited materials, especially in metals like tungsten, is critical in the development of new materials and the improvement of existing properties,” Tamm stated. “For example, such knowledge can be exploited in the development of more efficient and durable materials for a variety of applications, such as aviation, aerospace industry and energy production.”
In the study published in the journal Science Advances, physicists conducted experiments on a tungsten single-crystal sample. In such crystals, all atoms are arranged regularly throughout the crystal, resulting in a highly ordered structure. The scientists excited the crystal with ultra-short laser pulses. As a result, hot electrons reached temperatures of about 3000 degrees. These in turn affected the motion of ions by increasing their temperature. The goal of the scientists was to study this phenomena in detail using both experiments as well as atomistic computer modelling.
The beam of electrons passing a crystal will scatter, resulting in diffraction patterns that can reveal the position of the atoms. By analysing the time evolution of measured diffraction images, researchers can calculate how the atoms vibrate in a crystal at a picosecond time-scale. These time scales are barely even enough for light to penetrate through a window glass. The SLAC National Accelerator Laboratory in the United States has the capability to carry out such experiments and have already gained international recognition.
In the recently published study, scientists took a novel approach and were able to observe, for the first time, a the evolution of non-equilibrium lattice vibrations in a metallic system. According to the findings, laser-excited electrons initially affected high-frequency lattice vibrations in the tungsten crystal resulting in a non-uniform temperature distribution among them. Eventually the temperatures equilibrated and the entire material thermalised after approximately one hundred picoseconds.
Nothing like this has ever been observed in a metal anywhere in the world before. The observed non-equilibrium physical phenomenon requires strong interaction between electrons and ions, as well as the weak scattering of lattice vibrations in the crystal.
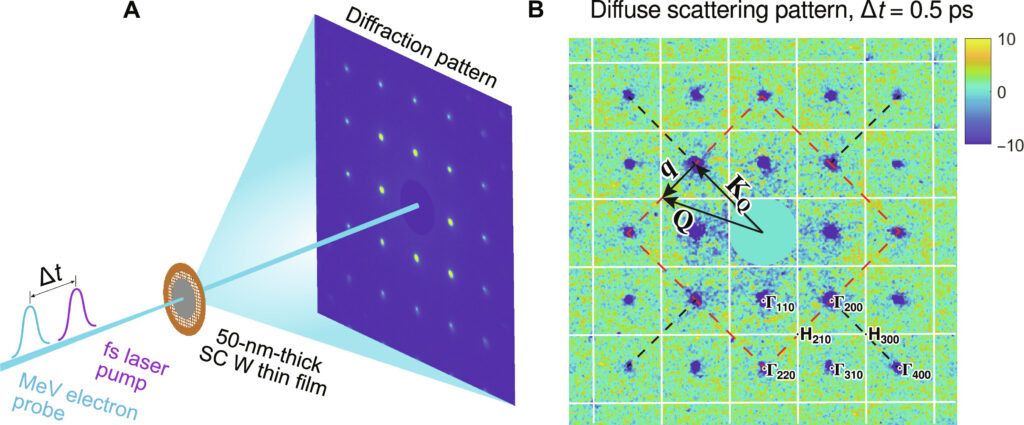
Firstly, a tungsten thin film is excited with a laser. A little while after, an electron beam is used to produce a diffraction pattern of the excited crystal. Adjusting the time delay allows for a series of images describing dynamics with picosecond resolution. Source: Science Advances
“Supercomputers designed for scientific simulations can model the same process at the atomic level,” noted Artur Tamm. “It is possible to generate an electron diffraction image based on the calculated atomic movements and compare it to the results of the experiment. The simulations are based on a novel model of ion-electron interaction developed by us.”
The associate professor explained that such experiments allow computers to play a larger role in the development of new materials. They help to validate that the models used by scientists describe the real-life occurrences correctly.
Moreover, the new theoretical model can be used to understand the results of experiments, as it is easy to extract more information from a simulation, and interpret the processes occurring in materials more accurately than before. This approach can also be used to verify the accuracy of models in complexly structured materials.
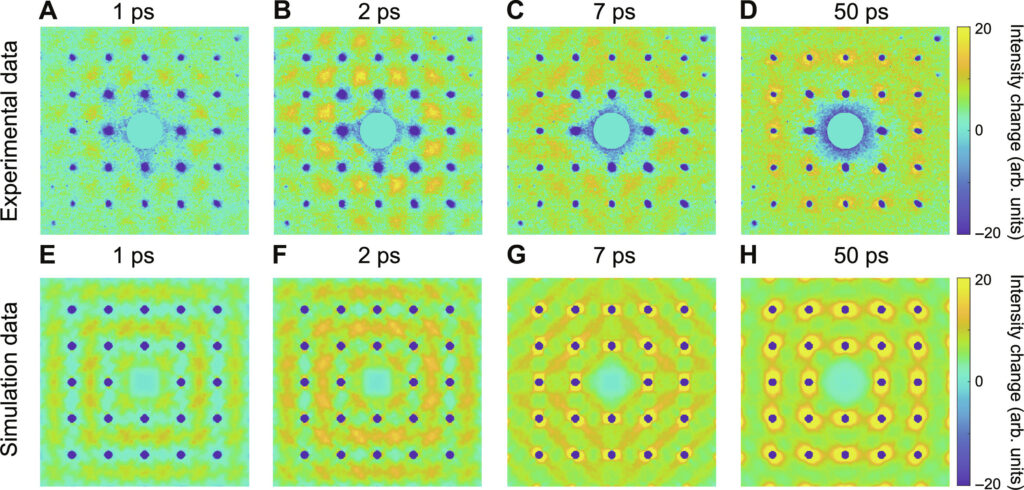
This modelling methodology has practical applications in studying materials used in nuclear reactors and evaluating their reliability and safety. This enables a better understanding of radiation-induced damage in materials and the development of more radiation-resistant materials, which can help minimise the damage caused by radiation.
Understanding nonequilibrium radiation damage processes is also important in the field of space technology. In the harsh conditions of outer space, electronic devices are exposed to high-energy particle fluxes and harsh radiation, both of which can be fatal. To mitigate risks, important components are usually duplicated, increasing the equipment’s mass. Thus, scientists are constantly looking for new materials and technologies that can withstand extreme conditions.
Understanding non-equilibrium radiation damage processes may also be important in other energy sectors, for example in the development of solar panels. This allows for a more accurate assessment of material durability and reliability under various conditions.
Tamm also stated that testing materials in extreme environments, such as in nuclear reactors and in space, is costly in labour and resources. “It also may not be possible to recreate the exact conditions that exist in reactors or in space,” said the physicist. “Therefore, material suitability for these applications often needs to be assessed from experiments by extrapolating the results, or instead materials are selected based on prior experience, which can stifle innovation. Computer simulations are a cost-effective method to test a large number of material types for specific applications and under conditions that mimic real-world environments.”
The associate professor stated that the biggest flaw in the calculations is the accuracy of the models. The more their accuracy is verified and accordingly fine-tuned, the better. “This study makes an important contribution to validating the methodology in the modelling of non-equilibrium processes and introduces a new tool for applied research and development,” said Tamm.
While one of the main objectives of the completed work was to demonstrate the usefulness of the approach in studying laser excitation of materials, further work will focus on processes involving high excitation energies as present in nuclear reactors.
This study was published in the journal Science Advances. In addition to Artur Tamm, another Estonian scientist involved in the experiment was Erki Metsanurk of Uppsala University.
This article was originally published on the Estonian Public Broadcasting online news portal. Editor: Jaan-Juhan Oidermaa
Read more about: What makes individuals fall through the safety nets during disasters?