Table of Contents
Despite widespread efforts to promote circular economy practices in response to the growing environmental crisis, vast amounts of organic waste still find their way into landfills. The key to transforming this waste into valuable resources might lie in the hands of tiny but powerful organisms like microbes and yeasts, which have the potential to turn what we discard into something valuable.
Each year, an average person living in the European Union generates around 513 kilograms of municipal waste. More than half of it ends up in a landfill [1]. This buried waste contains a significant amount of undecomposed resources that scientists refer to as the organic fraction of municipal solid waste. With some extra effort, two main organic streams could be actually used and processed into new products. The first organic stream is food residues and the second is lignocellulosic biomass consisting of grass, flower trimmings and yard waste [2].
Currently, household waste is primarily managed through methods like recycling and composting. The organic fraction can also be burned, which requires significant amounts of energy and creates additional greenhouse gas emissions. Another well-developed technology, “waste-to-energy”, converts non-recyclable residues into usable fuel, heat or electricity [3]. This technology still only reaches the first tier of the bio-based value pyramid, achievable with the organic fraction, which represents the lowest possible value of the products that could be created (Figure 1).
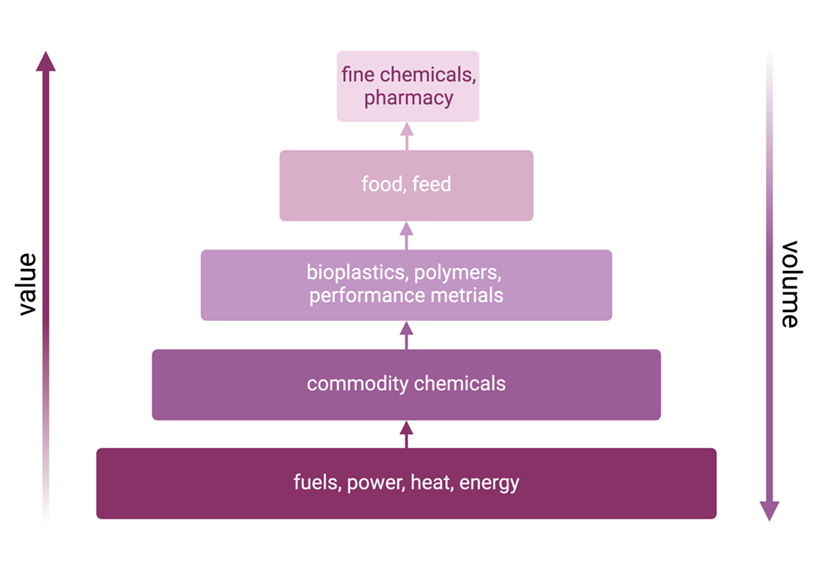
Virtues of decomposition
It is worth highlighting that although kitchen waste is not suitable for human consumption, it contains fats, proteins and carbohydrates that are a good feedstock for bacteria. We can obtain energy from it by using a mixture of anaerobic microorganisms that grow without oxygen. Through anaerobic digestion, we can convert complex organic matter into biomethane in four steps [4].
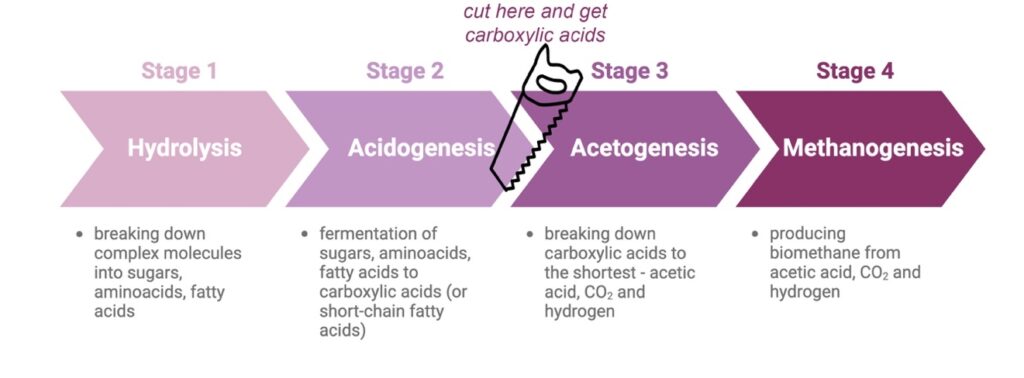
By omitting the last two steps, we can advance to the second tier of the bio-based value pyramid, focusing on the production of commodity chemicals. This process produces carboxylic acids, with the market price of 450-2800 € per ton (Figures 2 and 3). Scientists can make a significant amount of these acids by changing conditions like temperature, acidity, available nutrients and salinity. This also allows them to inhibit the production of methane and acetic acids.
Lignocellulosic biomass, such as yard waste, can also be broken down into smaller molecules using strong chemicals, but in only one step – hydrolysis. This process yields lignin and sugars, primarily xylose – the main sugar in hydrolysates. However, the sugar fraction is not completely pure and contains acids and furfurals – colorless, oily liquids – that most of the microorganisms don’t favor.
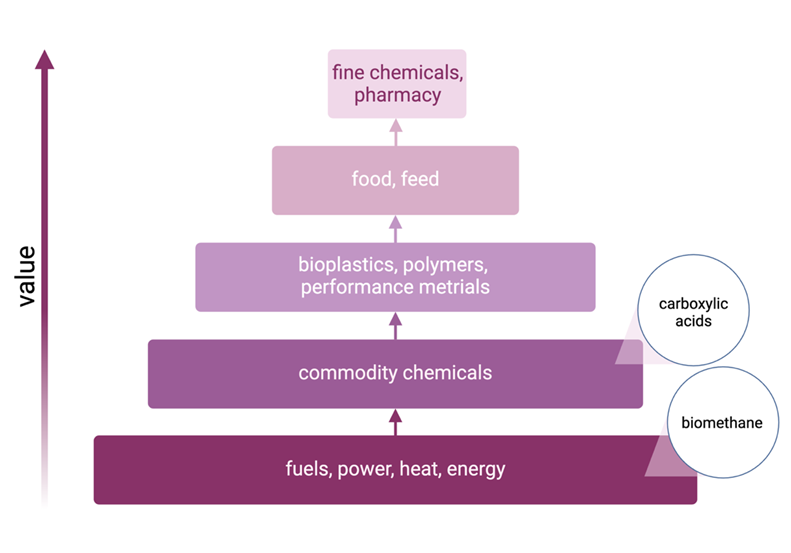
Increasing complexity
Now that we have commodity chemicals, it is worth aiming higher on the value pyramid. This requires introducing one more biotechnological process – using a pure culture of yeast that grows in the presence of oxygen, unlike bacteria in previous step. Cultivating a pure culture, however, requires sterility to avoid contamination by other microorganisms, which makes the process more laborious and expensive.
It is also challenging to find a yeast that can tolerate the leftover chemicals from lignocellulosic hydrolysis, consume xylose and grow on carboxylic acids produced during anaerobic fermentation. However, Rhodotorula toruloides, a yeast capable of performing these feats, does exist. During its growth, it produces microbial lipids and carotenoids, which are not price-competitive with petroleum diesel [6]. According to the value pyramid, fuel is also not a high-value product. However, oils can serve as raw materials to produce acrylate prepolymers and UV-curable coatings [7].
However, accumulating significant amounts of microbial oils requires limiting nitrogen and phosphorus in the nutrient media, which creates additional challenges for nitrogen and phosphorus removal from available sidestreams. Coupling the low to medium value of microbial oil products with these additional operational costs make this biotechnological route less desirable. So instead, we focus on the production of the carotenoids in R. toruloides.
The most abundant carotenoid in this yeast is beta-carotene, which, as the name suggest, is also found in carrots, giving them their orange color. β-carotene is valuable as a precursor of vitamin A (retinol), which is essential for eye health, vision, skin, and the immune system.
Additionally, it has antioxidant properties and may slow down cognitive decline [8]. Beta-carotene is used in food and feed, dietary supplements, personal care, and cosmetics. Currently, it is isolated from natural sources as well as produced by chemical synthesis [9]. Market price of β-carotene varies from 300 to 3000 USD per kg [10] (Figure 4).
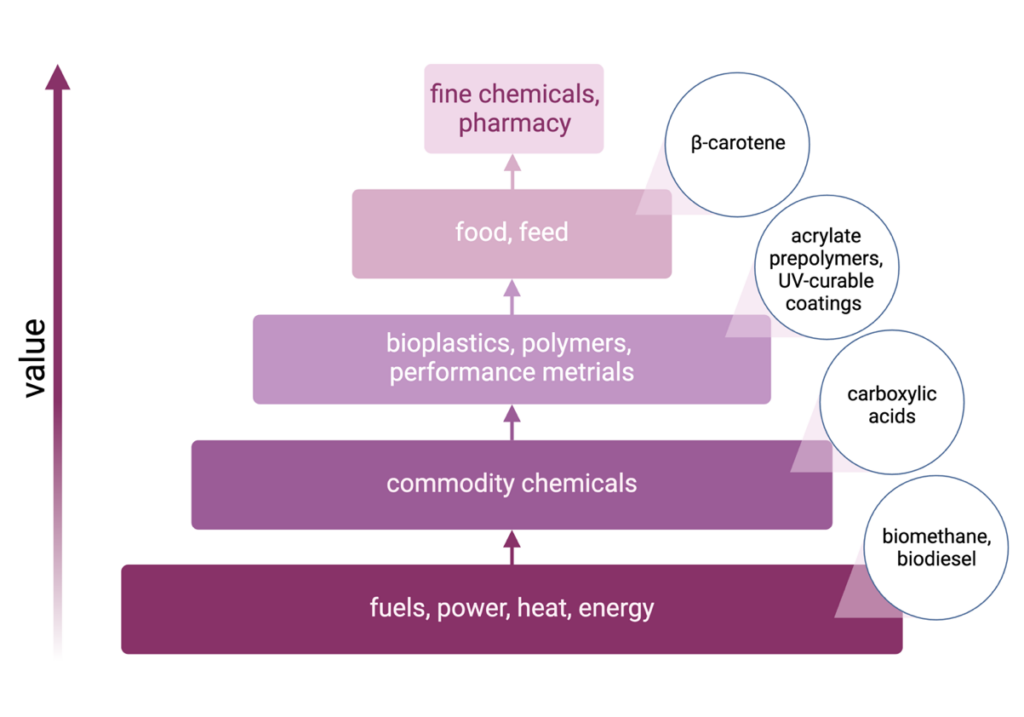
Increasing the value
Finding a suitable microorganism that thrives on sidestreams while producing something valuable is a complex task. Microorganisms found in nature usually produce desirable products in low quantities. Establishing biotechnological production requires further scientific research and development. Once in the laboratory, however, our goal is to produce astaxanthin, the most valuable carotenoid.
At this stage, we don’t introduce an additional biotechnological step but instead use genetic engineering tools to modify the previously mentioned yeast Rhodotorula toruloides. Instead of producing beta-carotene, in this case we aim to produce astaxanthin. To achieve this, we take a wild yeast from nature and fortify it with two genes, copied from algae that naturally produce astaxanthin. If we successfully integrate these genes into the yeast genome, we can convert β-carotene into astaxanthin within the cell (Figure 5).
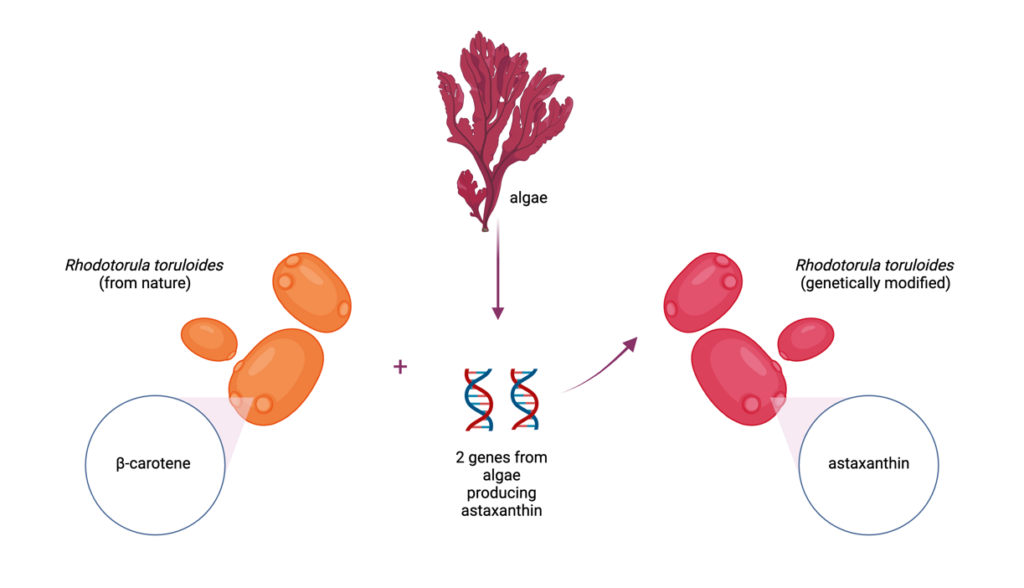
Astaxanthin is a red carotenoid with a market price from 2500 to 10,000 USD per kg [10]. It has stronger antioxidant properties than β-carotene, and is used in the aquaculture and poultry industries [11], as a supplement and in cosmetics [10]. The same molecule gives salmon its appealing red hue.
Recently, few clinical trials have explored the use of astaxanthin in preventing diseases related to oxidative stress, such as acute and chronic inflammatory conditions, metabolic diseases, cardiovascular diseases, central nervous system disorders, intestinal health conditions, and even improving exercise and physical performance [11].
Preliminary results are promising, highlighting additional potential benefits of astaxanthin. The astaxanthin available on a market today is mostly synthesized chemically, with some sourced from algae. Using genetically modified yeast can help move us towards sustainability while climbing to the top of the bio-based value pyramid. Once we do reach the top, we can close the carbon loop (Figure 6).
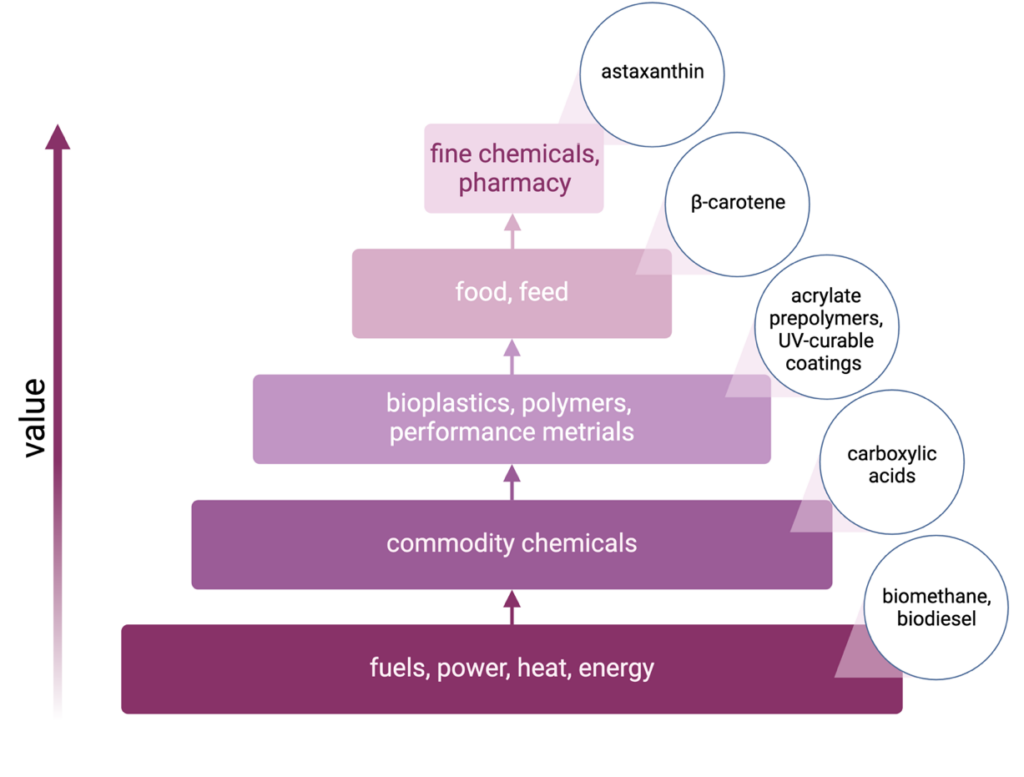
Closing the loop
These days, we often talk about the transition from linear to a circular economy. The linear economy model has left us with the problems of waste in landfills, excessive greenhouse gases emissions, and global pollution. From a biological perspective, however, circularity is a natural and logical process that has always existed on our planet. A good example of this is the carbon cycle (Figure 7).
Carbon is not meant to be stored in the landfill in undecomposed form. What scientists aim do in shifting from a linear economy to a circular one is simply to embrace the natural laws. By combining existing resources, we can move from the organic fraction of municipal solid waste to producing the most valuable carotenoids. This demonstrates how far we can go with recent advances in biotechnology and molecular biology.
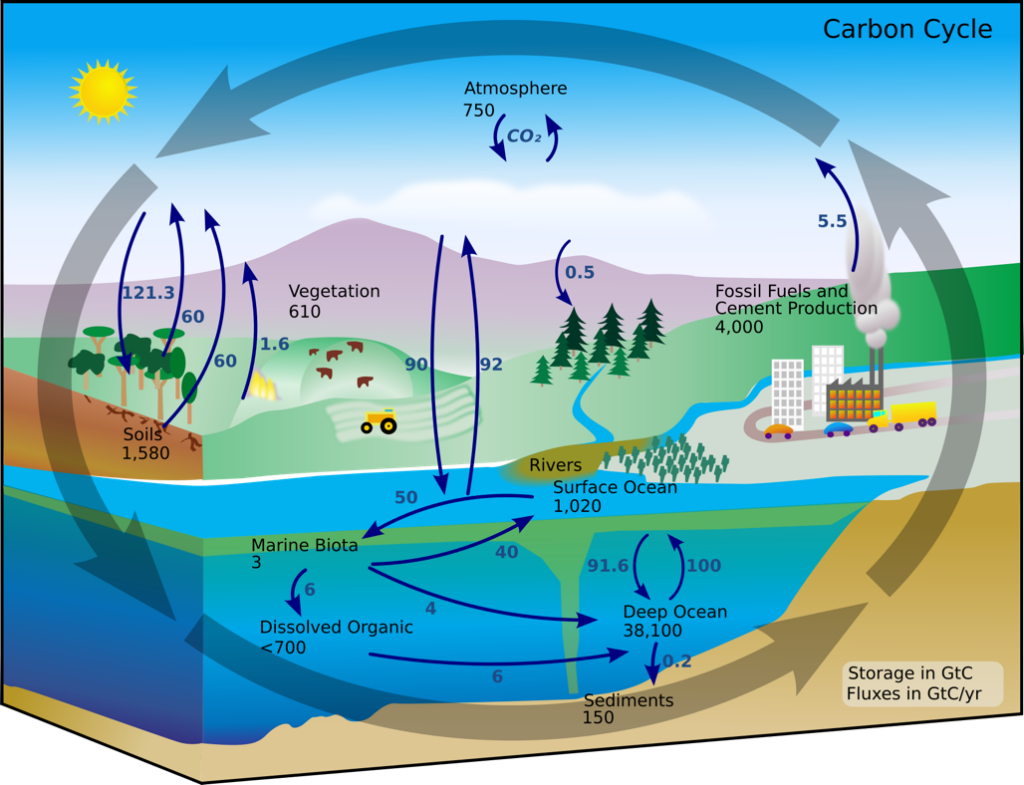
Acknowledgements
Thanks to Kaisa Orgusaar for reviewing the first draft of the article.
Author of this article is Inna Lipova. Editor Jaan-Juhan Oidermaa. Article written for the contest “Science in 3 minutes” organised by Estonian Academy of Sciences and The Estonian Young Academy of Sciences (EYAS). Main sponsor of the competition is Inclusive Financial Technology Foundation.
References
1. Eurostat: Municipal waste statistics / https://ec.europa.eu/eurostat/statistics-explained/index.php?title=Municipal_waste_statistics
2. Organic Fraction of Municipal Solid Waste: Overview of Treatment Methodologies to Enhance Anaerobic Biodegradability / https://doi.org/10.3389/fenrg.2018.00075
3. Municipal Solid Waste Management and Disposal / https://www.ijert.org/municipal-solid-waste-management-and-disposal
4. Anaerobic digestion / https://www.e-education.psu.edu/egee439/node/727
5. Complex Effluent Streams as a Potential Source of Volatile Fatty Acids / https://doi.org/10.1007/s12649-013-9202-6
6. Rhodotorula toruloides: an ideal microbial cell factory to produce oleochemicals, carotenoids, and other products / https://doi.org/10.1007/s11274-021-03201-4
7. One-Step Synthesis of Novel Renewable Vegetable Oil-Based Acrylate Prepolymers and Their Application in UV-Curable Coatings / https://doi.org/10.3390/polym12051165
8. All you need to know about beta carotene / https://www.medicalnewstoday.com/articles/252758
9. Beta-carotene Market Size & Trends / https://www.grandviewresearch.com/industry-analysis/beta-carotene-market
10. Commercialization and Marketing Potential of Carotenoids / http://dx.doi.org/10.1007/978-3-030-46459-2_27
11. Astaxanthin: Past, Present, and Future / https://doi.org/10.3390/md21100514
If this dive into the world of waste has got you thinking, don’t throw in the towel just yet—recycle your curiosity and head over to our next article on innovative ways we’re turning trash into treasure! Read more about how TalTech’s Chemists Leading the Charge in Green Chemistry.